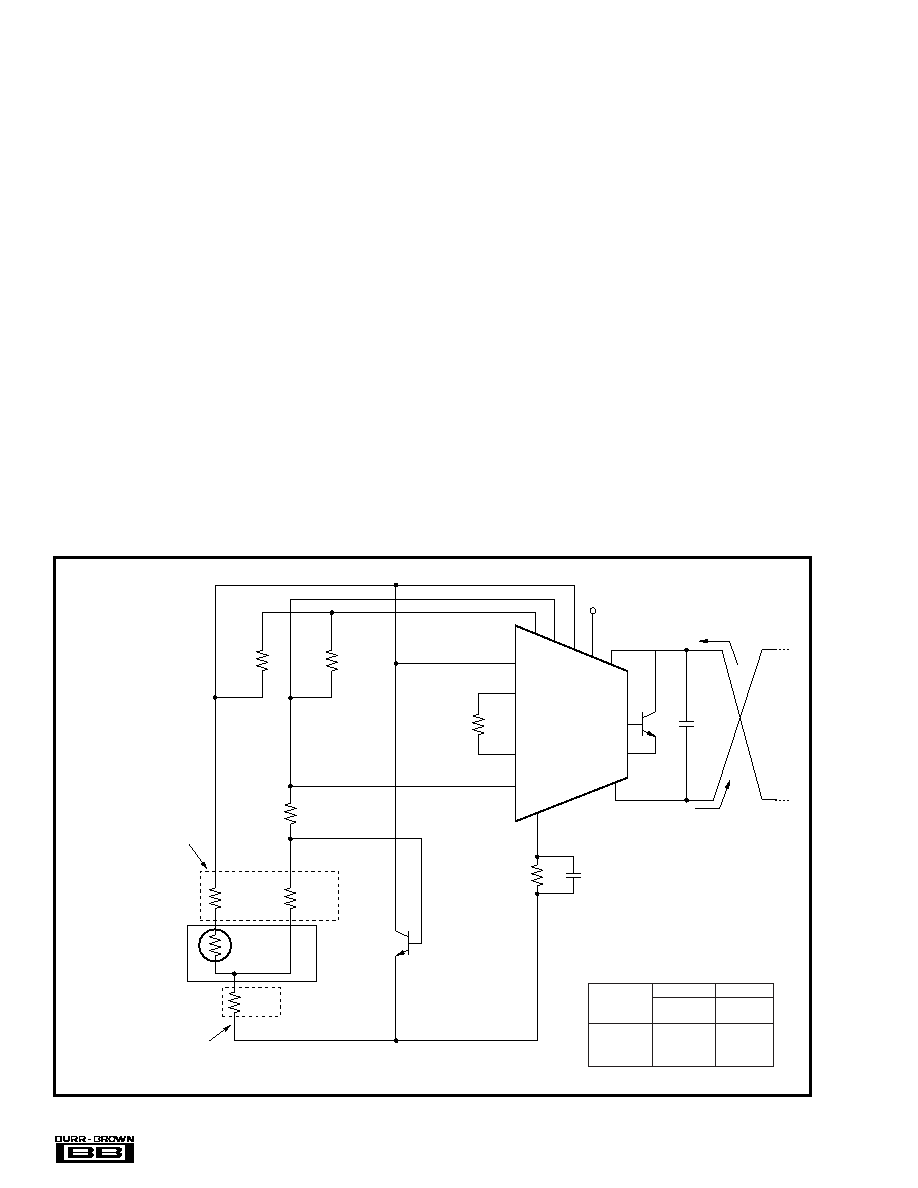
12
XTR112, XTR114
A typical two-wire RTD application with linearization is
shown in Figure 1. Resistor RLIN1 provides positive feed-
back and controls linearity correction. RLIN1 is chosen ac-
cording to the desired temperature range. An equation is
given in Figure 1.
In three-wire RTD connections, an additional resistor, RLIN2,
is required. As with the two-wire RTD application, RLIN1
provides positive feedback for linearization. RLIN2 provides
an offset canceling current to compensate for wiring resis-
tance encountered in remotely located RTDs. RLIN1 and RLIN2
are chosen such that their currents are equal. This makes the
voltage drop in the wiring resistance to the RTD a common-
mode signal which is rejected by the XTR112 and XTR114.
The nearest standard 1% resistor values for RLIN1 and RLIN2
should be adequate for most applications. Tables II and III
provide the 1% resistor values for a three-wire Pt1000 RTD
connection.
If no linearity correction is desired, the VLIN pin should be
left open. With no linearization, RG = 2500 VFS, where
VFS = full-scale input range.
RTDs
The text and figures thus far have assumed a Pt1000 RTD.
With higher resistance RTDs, the temperature range and
input voltage variation should be evaluated to ensure proper
common-mode biasing of the inputs. As mentioned earlier,
RCM can be adjusted to provide an additional voltage drop to
bias the inputs of the XTR112 and XTR114 within their
common-mode input range.
ERROR ANALYSIS
Table IV shows how to calculate the effect various error
sources have on circuit accuracy. A sample error calculation
for a typical RTD measurement circuit (Pt1000 RTD, 200
°C
measurement span) is provided. The results reveal the
XTR112’s and XTR114’s excellent accuracy, in this case 1%
unadjusted for the XTR112, 1.16% for the XTR114. Adjusting
resistors RG and RZ for gain and offset errors improves the
XTR112’s accuracy to 0.28% (0.31% for the XTR114). Note
that these are worst-case errors; guaranteed maximum values
were used in the calculations and all errors were assumed to be
positive (additive). The XTR112 and XTR114 achieve perfor-
mance which is difficult to obtain with discrete circuitry and
requires less space.
OPEN-CIRCUIT PROTECTION
The optional transistor Q2 in Figure 3 provides predictable
behavior with open-circuit RTD connections. It assures that if
any one of the three RTD connections is broken, the XTR’s
output current will go to either its high current limit (
≈ 27mA)
or low current limit (
≈ 1.3mA for XTR112 and ≈ 1mA for
XTR114). This is easily detected as an out-of-range condition.
FIGURE 3. Three-Wire Connection for Remotely Located RTDs.
I
O
I
O
XTR112
XTR114
OPEN RTD
TERMINAL
1
≈ 1.3mA
≈ 1mA
2
≈ 27mA
3
≈ 1.3mA
≈ 1mA
RTD
(R
LINE2)(RLINE1)
R
Z
(1)
R
LIN2
(1)
R
LIN1
(1)
(R
LINE3)
2
1
3
0.01
F
R
CM
0.01
F
Q
2
(2)
2N2222
NOTES: (1) See Table I for resistor equations and
1% values. (2) Q
2 optional. Provides predictable
output current if any one RTD connection is
broken:
13
4
3
2
R
G
XTR112
XTR114
7
6
(1)
R
G
R
G
V
IN
–
V
IN
+
V
LIN I
R1
I
R2
V
REG
V+
I
RET
I
O
E
B
8
9
Q
1
I
O
I
O
14
11
12
1
10
EQUAL line resistances here
creates a small common-mode
voltage which is rejected by
XTR112 and XTR114.
Resistance in this line causes
a small common-mode voltage
which is rejected by XTR112
and XTR114.