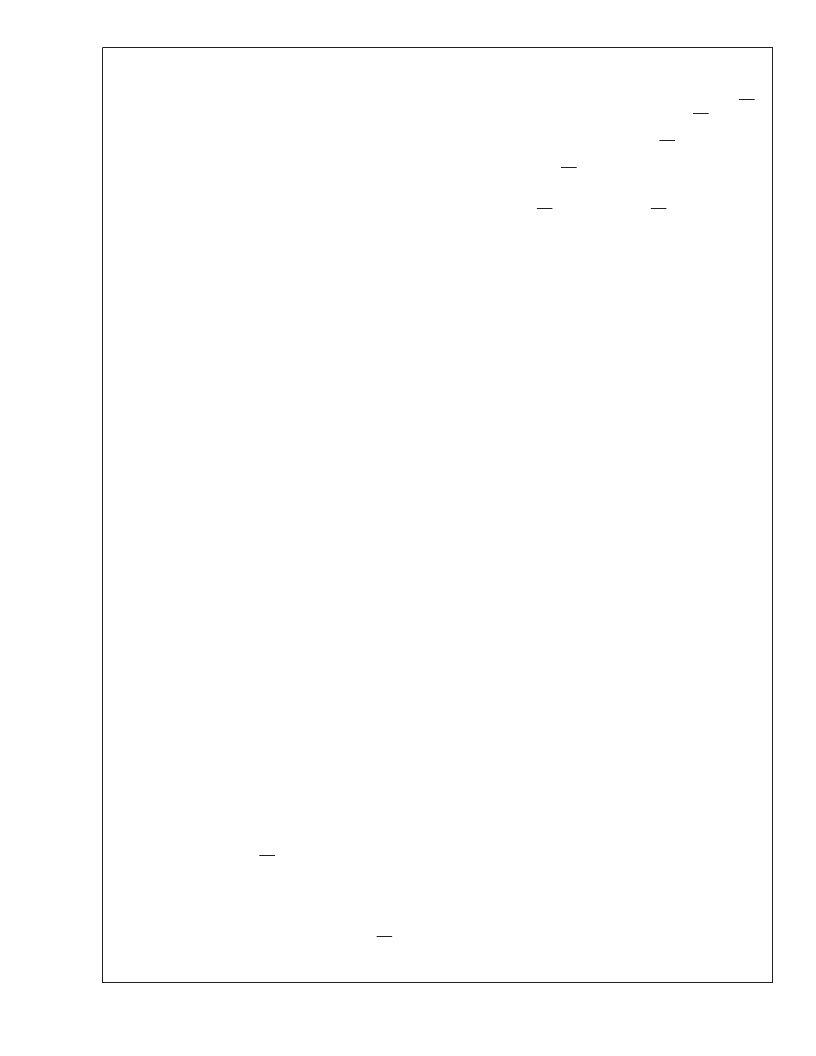
Application Information
(Continued)
Since the LM4953 has two operational amplifiers in one
package, the maximum internal power dissipation point is
twice that of the number which results from Equation 1. Even
with large internal power dissipation, the LM4953 does not
require heat sinking over a large range of ambient tempera-
tures. The maximum power dissipation point obtained must
not be greater than the power dissipation that results from
Equation 2:
P
DMAX
= (T
JMAX
- T
A
) / (
θ
JA
)
(2)
Depending on the ambient temperature, T
, of the system
surroundings, Equation 2 can be used to find the maximum
internal power dissipation supported by the IC packaging. If
the result of Equation 1 is greater than that of Equation 2,
then either the supply voltage must be decreased, the load
impedance increased or T
reduced. Power dissipation is a
function of output power and thus, if typical operation is not
around the maximum power dissipation point, the ambient
temperature may be increased accordingly.
POWER SUPPLY BYPASSING
As with any power amplifier, proper supply bypassing is
critical for low noise performance and high power supply
rejection. Applications that employ a 3V power supply typi-
cally use a 4.7μF capacitor in parallel with a 0.1μF ceramic
filter capacitor to stabilize the power supply’s output, reduce
noise on the supply line, and improve the supply’s transient
response. Keep the length of leads and traces that connect
capacitors between the LM4953’s power supply pin and
ground as short as possible.
AUTOMATIC STANDBY MODE
The LM4953 features Automatic Standby Mode circuitry
(patent pending). In the absence of an input signal, after
approximately 3 seconds, the LM4953 goes into low current
standby mode. The LM4953 recovers into full power operat-
ing mode immediately after a signal, which is greater than
the input threshold voltage, is applied to either the left or right
input pins. The input threshold voltage is not a static value,
as the supply voltage increases, the input threshold voltage
decreases. This feature reduces power supply current con-
sumption in battery operated applications.
To ensure correct operation of Automatic Standby Mode,
proper layout techniques should be implemented. Separat-
ing PGND and SGND can help reduce noise entering the
LM4953 in noisy environments. It is also important to use
correct power off sequencing. The device should be in shut-
down and then powered off in order to ensure proper func-
tionality of the Auto-Standby feature. While Automatic
Standby Mode reduces power consumption very effectively
during silent periods, maximum power saving is achieved by
putting the device into shutdown when it is not in use.
MICRO POWER SHUTDOWN
The voltage applied to the SD controls the LM4953’s shut-
down function. When active, the LM4953’s micropower shut-
down feature turns off the amplifiers’ bias circuitry, reducing
the supply current. The trigger point is 0.3*CPV
for a
logic-low level, and 0.7*CPV
for logic-high level. The low
0.01μA (typ) shutdown current is achieved by applying a
voltage that is as near as ground a possible to the SD pins.
A voltage that is higher than ground may increase the shut-
down current.
There are a few ways to control the micro-power shutdown.
These include using a single-pole, single-throw switch, a
microprocessor, or a microcontroller. When using a switch,
connect an external 100k
pull-up resistor between the SD
pins and V
. Connect the switch between the SD pins and
ground. Select normal amplifier operation by opening the
switch. Closing the switch connects the SD pins to ground,
activating micro-power shutdown. The switch and resistor
guarantee that the SD pins will not float. This prevents
unwanted state changes. In a system with a microprocessor
or microcontroller, use a digital output to apply the control
voltage to the SD pins. Driving the SD pins with active
circuitry eliminates the pull-up resistor.
SELECTING PROPER EXTERNAL COMPONENTS
Optimizing the LM4953’s performance requires properly se-
lecting external components. Though the LM4953 operates
well when using external components with wide tolerances,
best performance is achieved by optimizing component val-
ues.
Charge Pump Capacitor Selection
Use low ESR (equivalent series resistance) (
<
100m
) ce-
ramic capacitors with an X7R dielectric for best perfor-
mance. Low ESR capacitors keep the charge pump output
impedance to a minimum, extending the headroom on the
negative supply. Higher ESR capacitors result in reduced
output power from the audio amplifiers.
Charge pump load regulation and output impedance are
affected by the value of the flying capacitor (C1). A larger
valued C1 (up to 3.3uF) improves load regulation and mini-
mizes charge pump output resistance. Beyond 3.3uF, the
switch-on resistance dominates the output impedance for
capacitor values above 2.2uF.
The output ripple is affected by the value and ESR of the
output capacitor (C2). Larger capacitors reduce output ripple
on the negative power supply. Lower ESR capacitors mini-
mize the output ripple and reduce the output impedance of
the charge pump.
The LM4953 charge pump design is optimized for 2.2uF, low
ESR, ceramic, flying, and output capacitors.
Input Capacitor Value Selection
Amplifying the lowest audio frequencies requires high value
input coupling capacitors (C
in Figure 1). A high value ca-
pacitor can be expensive and may compromise space effi-
ciency in portable designs. In many cases, however, the
speakers used in portable systems, whether internal or ex-
ternal, have little ability to reproduce signals below 150Hz.
Applications using speakers with this limited frequency re-
sponse reap little improvement by using high value input and
output capacitors.
Besides affecting system cost and size, C
has an effect on
the LM4953’s click and pop performance. The magnitude of
the pop is directly proportional to the input capacitor’s size.
Thus, pops can be minimized by selecting an input capacitor
value that is no higher than necessary to meet the desired
3dB frequency.
As shown in Figure 1, the internal input resistor, R
i
and the
input capacitor, C
i
, produce a -3dB high pass filter cutoff
frequency that is found using Equation (3). Conventional
headphone amplifiers require output capacitors; Equation (3)
can be used, along with the value of R
L
, to determine to-
wards the value of output capacitor needed to produce a
–3dB high pass filter cutoff frequency.
L
www.national.com
8